HOW TO CHOOSE A CCD/CMOS CAMERA FOR YOUR SETUP
- Chris Willocks
- Apr 10, 2023
- 7 min read
Updated: Jun 16, 2023
Choosing a CCD or CMOS camera that matches your setup is vitally important and can mean the difference between mediocre images and APOD worthy gems.

With so many different astro-imaging cameras out there to choose from, it can be a minefield trying to decide what to buy. This is especially true for beginners, as the technical specs and jargon can be overwhelming. It might be tempting to go for the most expensive one that you can afford, however this might not provide the most optimal results. There are a range of different factors that must be considered before committing to a purchase, including whether the camera is suitable for your telescope or mount.
Specifically, some of the main factors that require consideration are:
Field-of-view (FOV).
Sampling.
Mount tracking accuracy.
Let's dive deeper into these, so that we can better understand them.
Field-of-view (FOV)
One of the first things to consider when looking to purchase a CCD or CMOS camera is whether you want to image large, wide-field objects, such as emission nebulae and open star clusters, or smaller objects such as planetary nebulae and galaxies? This decision can make quite a difference to the type of camera that you require.
Generally speaking, the field-of-view (FOV) is dependent on the focal length of your telescope and the dimensions of the sensor in the camera. More specifically, for a constant focal length, a camera with a larger sensor will cover more area of the sky than a smaller sensor and will therefore provide a larger FOV. When the camera isn't changed, a longer focal length will provide a smaller FOV and vice versa.
A useful online tool to use to determine what field-of-view a specific camera will provide is a field-of-view calculator, such as this one here. Tools such as these allow you to specify a certain telescope/camera combination and superimpose the camera field-of-view over a specific deep sky object. An example for the Pleiades (M45) is shown below with various scope/camera configurations:
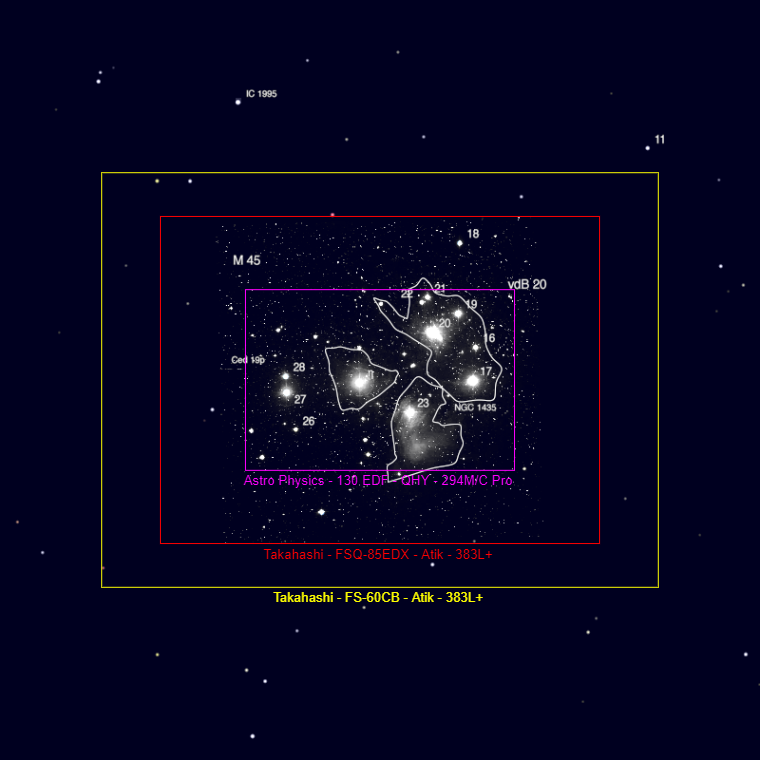
Field-of-view calculators allow you to select various different deep sky objects, such as large nebulae and small galaxies. This is useful in determining what objects you'll be able to image with your rig.
As mentioned above - the field-of-view is proportional to the size of the sensor when focal length remains the same. However, the overall size of the sensor is dependent on its resolution and pixel size. A higher resolution (more pixels) and larger pixel size will both produce a larger sensor and therefore a larger field-of-view.
Once you have determined the ideal field-of-view, along with what type of objects you would like to frame, now we need to consider sampling, which relates more to pixel size, as briefly touched on above.
Sampling
Sampling can be described in simple terms as how many pixels cover a specific area of the sky, such as a star. Sampling is determined by the combination of the pixel size of the camera sensor and the focal length of the telescope. We want the stars in our images to cover enough pixels in order to retain their round shape. The smallest pixel size that we can use is limited mainly by the atmospheric seeing of the imaging site. The better the seeing, the smaller the pixel size that we can use. This is generally why you see professional observatories on the top of mountains, where the atmosphere is thinner, allowing for better seeing. It allows for significantly higher resolution images. We refer to the area of sky each pixel covers as image scale or pixel scale. This is measured in arcseconds/pixel ("/px). This is an important term to remember. Image scale can also be limited by the Dawes' limit, when the aperture of the telescope is very small, but this rarely applies in reality, as seeing usually takes precedence well before.
If the pixel size of the camera is too large, then we won't have enough pixels covering a star, making the star appear blocky and unnatural. In other words, the resolution is too low. This is known as undersampling. Undersampling is more common when imaging with a short focal length telescope, such as a wide-field refractor. However, newer high-resolution cameras with very small pixels, such as CMOS cameras tend to solve this problem. Undersampling is not too much of an issue when using a wide-field telescope as the finest details are not usually that vital. Plus, post-processing techniques such as Drizzle can reduce the effects of undersampling. In fact, many APOD images are actually undersampled.
On the other hand, if the pixel size is too small, then we will have too many pixels covering a star. This will make stars look smoother, however can sometimes make them also look bloated. Longer exposure times are usually required, as smaller pixels do not capture as much light as larger pixels. This unnecessarily increases the required exposure time. This is known as oversampling. Also, the higher resolution can be limited by the atmospheric seeing and can put more demand on the tracking capabilities of your mount.
In the 1920s, Harold Nyquist derived the Nyquist theorem, which in basic terms suggests that the sampling rate of an analogue signal, should be double its frequency. However, because modern imaging cameras have square pixels and we want to image round stars, it is better to image with a resolution 1/3 of the analog signal,. Doing this will ensure a star will always fall on multiple pixels so remain circular.
Therefore, taking into account the above, if we assume that the average seeing for a typical imaging site is 2" to 4" FWHM, then the optimum camera pixel size or image scale would be between 0.67"/px and 2"/px (0.67 = 2 / 3, 2 = 4 / 2).
There are also online calculators that also determine the image scale for a specific telescope/camera combination, such as this one.
As we said, crudely speaking, the ideal image scale for average seeing is between 0.67"/px and 2"/px. However, the seeing can vary from night to night and from location to location. Therefore, it's important to have a rough idea of the seeing at your location each evening, so that you can determine whether the pixel scale of your camera is suitable. On some evenings when the seeing is particularly poor, it may be necessary to 'bin' your images. Binning essentially doubles or triples the pixel size of the camera, to make it more closely matched to the ideal sampling range, based on the seeing.
There are several pieces of software out there that can calculate actual seeing values for you at your site, based on a reference image that you provide, such as CCDInspector by CCDWare. Modern observatories tend to use more expensive devices called Differential Image Motion Monitors (DIMMs) to measure seeing, however these tend to be very expensive and are likely not very accessible to most amateurs.
Mount tracking accuracy
Mount tracking accuracy isn't specifically related to the camera but is very much worth mentioning at this point. Mounts are not perfect in terms of how accurately they track. Mechanical imperfections in the gears within the mount lead to what is known as Periodic Error (PE), which can give rise to tracking errors and trailing in long exposure images. It is usually measured through one complete revolution of the gear.
Periodic Error is more crucial when imaging at long focal lengths, as the effects of it are essentially magnified. This is because the image scale tends to be a lot smaller, which is less forgiving on tracking. This is one of the reasons why I would recommend that most beginners start off imaging with a short focal length refractor, as they generally offer larger image scales for a given camera, which is significantly more forgiving on your mount. It also means that a less expensive mount can be used. Long focal length scopes tend to be paired with expensive mounts, that have very low Periodic Error, such as the Paramount ME and 10 Micron GM series. Mounts such as the PlaneWave Ascension 200HR have what are known as high-resolution encoders, which essentially correct for any mechanical backlash or Periodic Error, giving the ability to take unguided long exposure images without any trailing.
Unfortunately, not everyone can afford such luxurious mounts as these. There are however ways in which you can reduce the effects of Periodic Error in your mount without spending a fortune:
Autoguiding - probably the easiest way of improving your images. An autoguider is a camera that makes sends correction signals to your mount's tracking by measuring the drift of star over a period of time. Note that high Periodic Error or backlash, as well as poor seeing can put added stress on your autoguider, making it less effective. Ideally, the guided (or unguided) Periodic Error RMS (Root Mean Square) of your mount should be half or less the value of your imaging camera's image scale, to provide the best results.
Adaptive Optics (AO) - some manufacturers including SBIG and Starlight Xpress offer what are known as Adaptive Optics units. Using the autoguider image to provide a reference image, these use an optical window that tilts quickly, to correct any shift in the image, rather than sending correction signals to the mount. They react significantly quicker than a mount and can be a great choice for less expensive mounts. One of the downsides is that they are known for being difficult to set up and can lead to much frustration in the hands of those that do not know how to use them properly.
Periodic Error Correction (PEC) - most modern mounts have this feature. Essentially, it allows you to train your mount to correct for Periodic Error by manually keeping a star centered in a high-power eyepiece over several gear cycles. The mount then learns the corrections and applies them to the tracking. This feature can be used in conjunction with autoguiding.
Mount tuning - some companies offer improved parts for your mount to reduce Periodic Error and backlash e.g. a belt drive system to replace a geared system. This can be a cheap and effective way of drastically improving your mount's performance.
Following the basic guidelines given above will allow you more time to focus on imaging and gaining valuable data, rather than spending hours trying to work out why your images don't quite look right.